Difference between revisions of "Research"
m (→Research) |
m (→Research) |
||
Line 11: | Line 11: | ||
To study these materials, our group makes use of femto-second coherent X-ray diffraction imaging to study ultra-fast SPT's in nanoscale materials. This process became possible with the advent of the X-ray Free Electron Laser (XFEL) synchrotron radiation facility. Here we combine Bragg coherent X-ray diffraction imaging (BCXDI) with a cross-correlated femto-second pump-probe scheme that allows us to image structural changes with a time resolution of the order of a few femto-seconds. BCXDI is a powerful lens-less imaging technique for probing crystalline materials with sub-nanometre sensitivity. When BCXDI imaging is performed using the femto-second timing of an XFEL, we can obtain three-dimensional images of nanoscale structures with femto-second temporal resolution and nanometre spatial resolution. As a result, we are able to address the fundamental problem of how to determine atomic motions during a SPT in solid-phase materials. Our findings will likely aid in the development of a dynamical theory that can fully describe this structural change. | To study these materials, our group makes use of femto-second coherent X-ray diffraction imaging to study ultra-fast SPT's in nanoscale materials. This process became possible with the advent of the X-ray Free Electron Laser (XFEL) synchrotron radiation facility. Here we combine Bragg coherent X-ray diffraction imaging (BCXDI) with a cross-correlated femto-second pump-probe scheme that allows us to image structural changes with a time resolution of the order of a few femto-seconds. BCXDI is a powerful lens-less imaging technique for probing crystalline materials with sub-nanometre sensitivity. When BCXDI imaging is performed using the femto-second timing of an XFEL, we can obtain three-dimensional images of nanoscale structures with femto-second temporal resolution and nanometre spatial resolution. As a result, we are able to address the fundamental problem of how to determine atomic motions during a SPT in solid-phase materials. Our findings will likely aid in the development of a dynamical theory that can fully describe this structural change. | ||
+ | |||
+ | === Ab Inito Molecular Dynamics === | ||
+ | |||
+ | [[File:md.mp4|thumb|right|500px|alt=Research Summary.|Supercell of VO<sub>2</sub> undergoing a structural solid-solid phase transition from monclinic to tetragonal structure.]] | ||
+ | |||
+ | Our group is also engaged in large scale supercomputer simulations of structural phase transitions. We make use of the Iridis high-performance computing facility to perform ab-initio molecular dynamics (MD) simulations of structural phase transitions under various conditions. Simulations of the ultra-fast SPT allow us to bridge XFEL experiments to current theory and to make predictions as to how the behaviour of a material changes under various conditions. This might relate to a change in the immediate environment of the material or the materials use in a heterostructure device setting. | ||
+ | |||
=== Strong Phase Structures === | === Strong Phase Structures === | ||
Line 18: | Line 25: | ||
Strain engineering plays an important role in the development of devices in the semiconductor industry today. It is also utilised directly in a number of device applications including piezoelectric actuators and sensors. When the amount of strain exceeds a certain limit (unique to each material), the accompanying strong phase structure makes strain mapping challenging. This research topic aims to investigate the origin of strong phase structures in nanometre scale materials and how they impact the CXD imaging process. We do so by systematically studying the transition into the strong phase structure regime. Results obtained here are also of value in developing advanced numerical optimisation techniques for phase recovery. | Strain engineering plays an important role in the development of devices in the semiconductor industry today. It is also utilised directly in a number of device applications including piezoelectric actuators and sensors. When the amount of strain exceeds a certain limit (unique to each material), the accompanying strong phase structure makes strain mapping challenging. This research topic aims to investigate the origin of strong phase structures in nanometre scale materials and how they impact the CXD imaging process. We do so by systematically studying the transition into the strong phase structure regime. Results obtained here are also of value in developing advanced numerical optimisation techniques for phase recovery. | ||
− | |||
+ | === Non-linear Phase Retrieval Optimisation === | ||
Correlated electronic materials that undergo a spontaneous crystalline deformation can experience a significant strain. This can pose a challenge when reconstructing and interpreting information obtained from CXD imaging. We have previously shown that materials exhibiting strong phase structure require special optimisation methods to reconstruct the real-space object. Our group has developed the first algorithm to reconstruct data known to have strong phase structure. There are however still significant developments necessary to discover robust algorithms that are universally effective. | Correlated electronic materials that undergo a spontaneous crystalline deformation can experience a significant strain. This can pose a challenge when reconstructing and interpreting information obtained from CXD imaging. We have previously shown that materials exhibiting strong phase structure require special optimisation methods to reconstruct the real-space object. Our group has developed the first algorithm to reconstruct data known to have strong phase structure. There are however still significant developments necessary to discover robust algorithms that are universally effective. |
Revision as of 16:24, 6 October 2015
Contents
Research
Correlated electronic materials are those which the interaction between the valence electrons can strongly influence the materials properties. They are interesting as their unique properties are of considerable utility for device physics, functional materials and the study of fundamental condensed matter physics. Recently, there has been renewed interest in materials that exhibit a solid-solid structural phase transition at a critical temperature. Some of these materials exhibit a reconstructive structural phase transition (SPT) at some critical temperature that occurs on the femto-second time scale. There is still a great deal that is not known about the dynamics of this phenomenon as the ability to probe ultra-fast transitions remains largely inaccessible to conventional techniques. An example is vanadium dioxide (VO2), where spontaneous (non-diffusion limited) atomic rearrangement occurs due to external excitation. Switching speeds on the femto-second timescale have been observed.
To study these materials, our group makes use of femto-second coherent X-ray diffraction imaging to study ultra-fast SPT's in nanoscale materials. This process became possible with the advent of the X-ray Free Electron Laser (XFEL) synchrotron radiation facility. Here we combine Bragg coherent X-ray diffraction imaging (BCXDI) with a cross-correlated femto-second pump-probe scheme that allows us to image structural changes with a time resolution of the order of a few femto-seconds. BCXDI is a powerful lens-less imaging technique for probing crystalline materials with sub-nanometre sensitivity. When BCXDI imaging is performed using the femto-second timing of an XFEL, we can obtain three-dimensional images of nanoscale structures with femto-second temporal resolution and nanometre spatial resolution. As a result, we are able to address the fundamental problem of how to determine atomic motions during a SPT in solid-phase materials. Our findings will likely aid in the development of a dynamical theory that can fully describe this structural change.
Ab Inito Molecular Dynamics
Our group is also engaged in large scale supercomputer simulations of structural phase transitions. We make use of the Iridis high-performance computing facility to perform ab-initio molecular dynamics (MD) simulations of structural phase transitions under various conditions. Simulations of the ultra-fast SPT allow us to bridge XFEL experiments to current theory and to make predictions as to how the behaviour of a material changes under various conditions. This might relate to a change in the immediate environment of the material or the materials use in a heterostructure device setting.
Strong Phase Structures
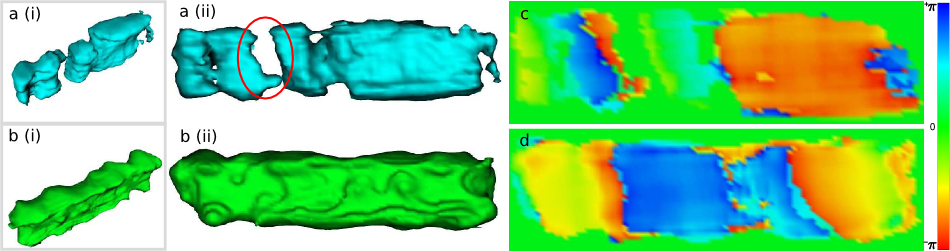
Strain engineering plays an important role in the development of devices in the semiconductor industry today. It is also utilised directly in a number of device applications including piezoelectric actuators and sensors. When the amount of strain exceeds a certain limit (unique to each material), the accompanying strong phase structure makes strain mapping challenging. This research topic aims to investigate the origin of strong phase structures in nanometre scale materials and how they impact the CXD imaging process. We do so by systematically studying the transition into the strong phase structure regime. Results obtained here are also of value in developing advanced numerical optimisation techniques for phase recovery.
Non-linear Phase Retrieval Optimisation
Correlated electronic materials that undergo a spontaneous crystalline deformation can experience a significant strain. This can pose a challenge when reconstructing and interpreting information obtained from CXD imaging. We have previously shown that materials exhibiting strong phase structure require special optimisation methods to reconstruct the real-space object. Our group has developed the first algorithm to reconstruct data known to have strong phase structure. There are however still significant developments necessary to discover robust algorithms that are universally effective.
Nanomaterials and Device
The increasing need to make smaller device structures with enhanced functionality has led the move away from conventional top-down lithographic approaches toward the utilisation of self-assembled nanoscale structures. The unique morphologies exhibited by many nanocrystals make them ideal for various applications including biochemical sensors, photodetectors, light emitters, transistors, high frequency oscillators, and waveguides. Our research into nanomaterials and devices is centred on self-assembled metal-oxide nanocrystals which have the advantage of high chemical resistance and low thermal degradance. This includes group II-VI semiconductor materials such as zinc oxide (ZnO).